- lauraclark849
- Mar 27, 2024
- 8 min read
Updated: Apr 22, 2024
Peer Review Article | Open Access | Published 3rd April 2024
A comparative study of a standard slit-to-agar sampler and a real-time bacterial detector
Bengt Ljungqvist¹, Johan Nordenadler ², Berit Reinmüller ¹ | EJPPS | 291 (2024) | https://doi.org/10.37521/ejpps.29102 | Click to download pdf
Keywords: Airborne particles, controlled environment, CFU, fluorescent particles, real-time measurements, viable particles.
ABSTRACT
A comparison is presented of data from simultaneous measurements of airborne particles by a standard slit-to-agar sampler (STA) and of fluorescent particles by a real-time bacterial detector, Bio Aerosol Monitoring System (BAMS) during an evaluation regarding emitted airborne particles of a surgical clothing system in a test chamber. Data from simultaneous orientating measurements of airborne particles by a standard discrete particle counter are also discussed.
The results show that in an environment with a low level of airborne particles including CFUs and man the only contamination source, there is a moderate correlation between the number of aerobic CFU and BAMS viable, i.e., fluorescence, particles, when the slit-to-agar sampler is registering/collecting within its detection level.
Different environments need simultaneous measurements by an STA in aerobic CFU/m³ and by a BAMS in viable particles/m³ to set up the correlation between the two methods.
INTRODUCTION
Orthopedic surgery is usually classified as infection-prone surgery and mostly performed in ultraclean air operating rooms. Thus, maintaining a high-level of microbial air cleanliness (≤10 CFU/m³) in the operating room is of high importance, see e.g., SIS TS 39:2015¹ and Whyte, Lytsy².
The present guidelines for environmental microbial monitoring of air in the pharmaceutical industry (≤10 CFU/m³) are provided in GMP guidance documents published by the EU and FDA³,⁴.
Ultraclean air operating rooms for infection-prone surgery in hospitals and premises for aseptic production of sterile pharmaceutical products have demands/requirements on high microbial air cleanliness during activity, both with a mean value of less than or equal to 10 CFU per m³.
Real time monitoring has a great advantage over conventional monitoring because deviations can be detected immediately.
During conditions with very low concentrations of airborne viable particles, a simultaneous measuring study has been performed in a test chamber to compare the recoveries between a standard slit-to-agar sampler (STA), and Bio Aerosol Monitoring System (BAMS). Simultaneously orientating measurements were also performed with a standard discrete particle counter (DPC).
The Bio Aerosol Measuring System uses light scattering measurement according to the Mie scattering principle to detect, quantify and measure the sizes of airborne particles. The BAMS also uses laser induced fluorescence, which indicates the biological activity of the airborne particles to determine if they are microbial or inert particles. The emitted fluorescence is measured using a photodetector. The presence or absence of this fluorescent light is a direct indication of biological particles. These fluorescent particles are often called viables.
Materials and Methods
Monitoring equipment
In the exhaust duct of the test cabin, particles were collected on agar plates and detected as CFUs by using a STA (FH3™)⁵,⁶ with a d₅₀-value of 1.6µm, the total number of inert and viable particles, respectively, were sized and counted by BAMS. Orienting tests of total number of airborne particles were sized and counted by a DPC (HiacRoyco 245). All instruments were operated according to the manufacturers’ instructions.
The characteristics of the monitoring equipment/instruments are shown in Table 1.
Table 1 The characteristics of the monitoring equipment/instruments

Microbial growth medium for the STA tests was sterile standard Tryptic Soy Agar (TSA) in 9cm Petri dishes. The TSA plates were incubated not less than 3 days at 32°C followed by not less than 2 days at room temperature (22°C ±2°), and the number of CFUs were counted.
Test Conditions
The authors have performed the comparison during evaluation of a surgical clothing system of cleanroom quality regarding the efficacy to retain particles from the test subjects in a test cabin with a dispersal chamber (body-box).
The principal arrangement of the test cabin and the sampling locations are shown in Figure 1.
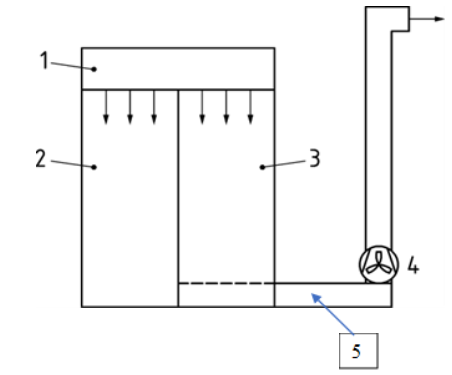
Figure 1. Principal arrangement of the test cabin with its changing area and the dispersal chamber (body-box). 1. HEPA-filter, 2. Changing area, 3. Test cabin (body box), 4. Exhaust fan, 5. Sampling location.
The test cabin was supplied with HEPA-filtered air (H14) with a vertical airflow. The concentration of airborne particles was measured in the exhaust duct through tubing connected to the measuring instruments, respectively. To establish the basic particle level of contamination in the empty chamber, samples were taken and the sampling period for all air samplers was set to 10 minutes.
With environmental conditions like those in GMP Grade A (≤1CFU/m³), the dispersal chamber was used for evaluation of a surgical clothing system of cleanroom quality. This study was conducted as described by Ljungqvist and Reinmüller⁷. The concentrations of viable particles, aerobic CFUs and airborne total particles were simultaneously measured in the exhaust air from the test chamber.
During the measurements, the test subject performed standardized cycles of movements including arm movements for 3 minutes, walk in place for 3 minutes, and knee bends for 3 minutes at a set speed. These movements are in principle comparable with those described in IEST-RP-CC003.4⁸. Prior to each test cycle of movement, the test subject stood still to avoid the influence of particle generation from the previous test cycle. The evaluated clothing system had five test subjects performing the three standardized cycles of movements four times. In total twenty cycles each of 10 minutes were conducted with the surgical clothing system of cleanroom quality.
Data analysis
The sample periods were 10 minutes and the concentrations in Tables 2 and 3 are given for STA in aerobic CFUs per m³, for BAMS in viable particles per m³, and the orienting tests for DPC in total number of particles per m³.
Evaluation of data is performed by simple linear regression and the correlation coefficients are calculated according to ordinary textbooks, see e.g., Biostatistics by Daniel⁹.
RESULTS
Measurements performed in the empty dispersal chamber showed no detection of aerobic CFU (STA), no viable particles (BAMS), and no total number of particles (DPC). Results of the concentration measurements are shown in Tables 2 and 3, where the correlation coefficients, (simple linear regression) are calculated for registered concentrations of STA, aerobic CFU and BAMS, viable particles. It should be noted that BAMS viable particles i.e., fluorescence particles, are registered as, ≥0.5µm, ≥1µm, ≥2µm, ≥3µm, ≥5µm, and ≥10µm.
Table 2. Concentrations of aerobic CFU/m³ (STA), and viable particles/m³ (BAMS) measured in the dispersal chamber, where five test subjects dressed in a surgical clothing system performed four standardized cycles of movements, i.e., in total 20 cycles.
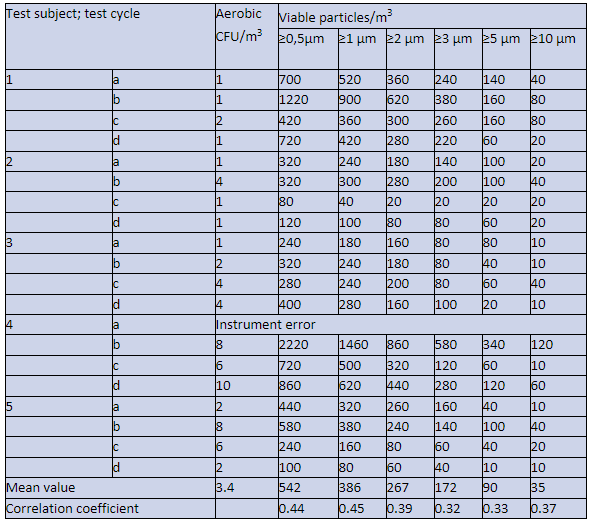
*Mean value of aerobic CFU/m³ is given with one decimal and mean value for viable particles/m³ are integer numbers.
The number of test cycles in Table 3 are reduced so all values for aerobic CFU/m³ measured with the STA are within the detection level (≥2 aerobic CFU/m3). In Table 3 are the total number of particles per m³ (DPC) given.
Table 3. Concentrations of aerobic CFU/m3 (STA), and viable particles/m³ (BAMS), and total number of particles/m³ (DPC) measured in a dispersal chamber, where five test subjects dressed in a surgical clothing system, performed four standardized cycles of movements. All values are within the instrument’s detection levels.
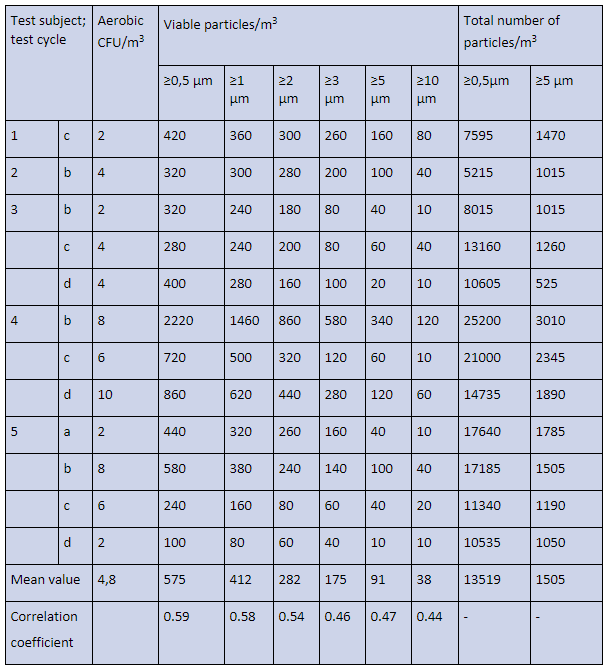
*Mean value of aerobic CFU/m³ is given with one decimal and mean values for viable particles/m³ are integer numbers.
When the linear correlation coefficient is around 0.4 there is a weak correlation, while when the correlation reaches 0.6 the correlation is moderate and starts to become acceptable, see e.g., Biostatistics by Daniel⁹.
The difference of correlation coefficients in Table 2 and Table 3 might be because some data in Table 2 are below the detection level of the STA, i.e., less than 2 aerobic CFU/m³, while in Table 3 all data are within the detection level of the STA.
It could also be noted that the correlation coefficient between CFUs and particles ≥0.5, ≥1, and ≥2 µm is higher than for the larger particle sizes. This could be explained by the efficiency of the tested clothing system to retain large particles from the test subjects.
Discussion and Conclusion
The relations between the total number of airborne particles and the number of aerobic CFU or viable particles are different depending on the measuring technique used.
In this study, when the total number of airborne particles/m³ ≥0,5µm and ≥5µm were counted by the DPC, and the aerobic CFU/m3 were counted by the STA, the relations are about 2 800:1 and 300:1 respectively. This can be compared to cleanroom clothing systems of pharmaceutical grade B quality, where the corresponding relation is for airborne particles/m³ ≥0,5µm and aerobic CFU/m³ about 1000:1, see Ljungqvist, Reinmüller ¹⁰. The pharmaceutical cleanroom clothing systems are more effective than surgical clothing system to retain particles emitted from man to the air.
The ratio between viable particles/m³ ≥0,5µm counted by BAMS and aerobic CFU/m³ counted by STA is about 120:1.
The difference in number/m³ between viable particles and aerobic CFU/m³ can be explained as not all viable particles give rise to an aerobic CFU on a standard medium. This result is expected.
In spite of the limited study, it can be stated that there is a moderate correlation between data from simultaneous measurements in clean controlled environments (at low contamination levels) for STA in aerobic CFU/m³ and for BAMS in viable (i.e., fluorescence) particles/m³.
The challenge is to connect the results from BAMS to the conventional old measuring methods. However, this relation is probably different for different kinds of environments, such as operating rooms, pharmaceutical Grade B cleanrooms, and cleanrooms in food industry.
Different environments need simultaneous measurements by STA in aerobic CFU/m³ and by BAMS in viable particles/m³ to establish alert and action levels. The selection of sampling locations and probe placements is important. When alert and action levels are determined, a definition of “significant deviation” should also be decided. When a significant deviation occurs, it would require that immediate action be taken.
Real time monitoring has a great advantage over conventional methods since corrective actions can be taken when deviations are found. The disadvantage is that microbiological contaminants are not yet possible to identify with real time monitoring.
ACKNOWLEDGEMENT
To Mr. Per Holmström, Zecon AB, Sweden for technical assistance concerning data treatment of viable particles from BAMS.
References
01. Swedish Standard Institute, SIS -TS39:2015, Microbiological cleanliness in the operating room – Preventing airborne contaminants – Guidance and fundamental requirements, Stockholm, Sweden, SIS:2015.
02. Whyte, W., Lytzy, B., Ultraclean air systems and the claim that laminar airflow systems fail to prevent deep infections after total joint arthroplasty, J. Hosp Infect. 2019: Sept 103 (1): e9-e15.
03. European Commission, The Rules Governing Medicinal Products in the European Union, Volume 4; EU Guidelines for Good Manufacturing Practice for Medicinal Products for Human and Veterinary Use, Annex 1 - Manufacture of Sterile Products, Brussels, Belgium, European Conimission:2022.
04. Food and Drug Administration, Guidance for Industry, Sterile Drug Products Produced by Aseptic Processing – Current Good Manufacturing Practice, Rockville, MD, USA: FDA: 2004.
05. Ljungqvist, B., Reinmüller, B., Active sampling of airborne viable particles in controlled environments; a comparative study of common instruments, EJPPS, 1998; 3: 59-62.
06. Ljungqvist, B., Reinmüller, B., Monitoring efficiency of microbiological impaction air samplers, EJPPS, 2008; 13: 93-97.
07.Ljungqvist, B., Reinmüller, B., Cleanroom Clothing Systems; People as a Contamination Source, PDA/DHI Publishing, LLC, River Grove, IL., ISBN 1-930114-60-5, 2004.
08. Institute of Environmental Sciences and Technology. IEST-RP-CC003.4 Garment System Considerations for Cleanrooms and Other Controlled Environments, Arlington Heights, IL., USA: IEST: 2011.
Authors
Bengt Ljungqvist ¹, Johan Nordenadler ² and Berit Reinmüller ¹
1 Building Services Engineering, Chalmers University of Technology, Göteborg,
Sweden.
2 CLINTEC, Karolinska Institute, Huddinge, Sweden.
Contact person:
Berit Reinmüller
e-mail beritr@chalmers.se
phone +46 (0)70 164 3411.

留言